Research Area C extends the length scale of interest from the molecular level to the nanoscale where collective effects play a defining role in material properties. The study of ultrafast ordering phenomena and nucleation events is not only crucial for understanding these materials but also for the development of tools for nanoscience.
With the new X-ray light sources ordering and nucleation can be investigated down to fundamental time scales of atom mobility in solids and solution, covering even short-living transient states. Research Area C is divided into three different Research Foci which focus all on time resolved investigations of ordering phenomena on the nanoscale. RFC.1 addresses the role of transient structures in molecular liquids such as water as well as the role of structural and orientational correlations for the glass transition. In RFC.2 we study nucleation and growth processes of nanoparticles and correlate their shape and phase transformations with external triggers. The subject of RFC.3 is the study of ultrafast spin ordering processes in nanostructures under the influence of dipolar and exchange interactions.
Research Focus C.1: Correlations and Dynamics in Disordered Model Systems
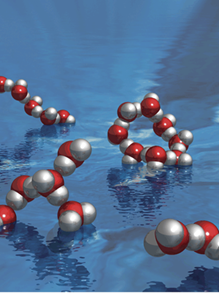
Snapshot of water structure [Y. Zubavicus and M. Grunze: Science 304, 974 (2004)]
In contrast to crystalline matter, disordered matter, such as liquids and glasses, do not exhibit long-range translational order but are able to accommodate various local structures. Among those, local icosahedral order has been suggested based on computer simulations. These symmetries may be of transient nature, exist only for a short time and are believed to be responsible for the supercooling of liquids and the existence of a glassy state. Although being in the focus of science for decades, little is known about the microscopic structure of liquids and glasses. This can be overcome by using the ultrashort and coherent X-ray pulses from hard X-ray FEL sources such as the Linac Coherent Light Source (LCLS) or the European XFEL. In RFC.1. we focus on the study of complex fluids with special emphasis on the question of (i) whether or not orientational order is responsible for glass transitions (RFC.1.1) and (ii) whether or not transient local structures can explain the peculiar behavior of complex fluids such as water (RFC.1.2). For both projects we develop and apply higher-order correlation functions in the framework of X-ray Cross Correlation Analysis (XCCA) to reveal such local structures.
RFC.1.1 focuses on the study of the structure and dynamics of glass-forming fluids. Colloidal fluids are important model systems for the physics of liquids and glasses because parameters such as density, size, and interaction potentials can be chosen at will. In particular, the whole phase diagram including the liquid, crystalline and glass phase can be easily accessed. In the framework of this project we test different scenarios for the glass transition by probing the local order from the liquid to the glassy phase.
In RFC.1.2 we study the structure of water and molecular fluids. Water, in particular, is suggested to accommodate various locally ordered structures that are believed to be responsible for water’s unique properties. Although different simulation and spectroscopy studies support the idea of local ordering a direct experimental proof is missing. With the advent of hard X-ray FEL sources it is possible for the first time to take snapshots of the instantaneous structure of water by XCCA. Besides water, local structural correlations such as icosahedrally ordered clusters are proposed for simple monoatomic liquids, e.g. liquid metals. As liquid metals scatter X-rays strongly they are ideal candidates to apply the concept of XCCA for the first time down to atomic length scales.
Research Focus C.2: Structure Formation in Nanomaterials
The usage of functional nanomaterials in medicine, engineering, energy and information technology heavily relies on our ability to control their formation and properties on an atomic level. Very small nanocrystals exhibit atomically defined structures with molecular orbitals governing the electronic and optical properties. When going to larger nanomaterials a transition/crossover to bulk properties and continuous electronic bands will show up. In this research focus we look into the structural formation and growth and into the steered transformation of nanomaterials. We unravel the associated dynamics and kinetics on an atomic level of detail by using X-ray light sources and spectroscopic methods which allow us to monitor the corresponding processes on the relevant time scales of atomic motions. These activities are methodically related to the pump-probe techniques of research area B and the cross correlation methods from research focus C.1. The acquired knowledge will eventually enable us to atomically control matter on the mesoscale and to tailor electronic, optical, magnetic and mechanic properties.
We follow two major directions:
- Firstly, we monitor and analyze early nucleation events leading to the formation of local atomic order and reaction intermediates. Whereas later stages of nucleation and growth can be described by continuum theories involving macroscopic quantities like the surface tension the understanding of the formation and characteristics of transient local order during the initial stage requires an atomic viewpoint. Our experiments with metal and semiconductor nanosystems with atomic resolution are therefore accompanied by the development of suitable atomistic models and associated theoretical studies of the dynamics and kinetics of early nucleation events. Further questions we are interested in are centered around the influence of surface capping ligands on nanocluster properties as well as nucleation and growth phenomena in confined geometries such as nanoporous matrices.
- In the second major thread we follow deals with shape and phase transitions of nanoparticles. In particular, we set focus on the dynamics of melting and subsequent recrystallization processes which we tackle with X-ray scattering and optical spectroscopy. In contrast to bulk transitions and their typical stochastic solid-state behaviour phase transitions in nanocrystals are usually governed by single particle events so that the adjunctive structural changes are fully reversible. Nanoparticles represent therefore ideal model systems to study the characteristic mechanisms of externally triggered phase transitions in different environments (e.g. in solutions, on substrates, in confined spaces) on an atomic level. Furthermore, we pay special attention to spatially and time resolved investigations of melting mechanisms in nano-heterojunctions of well-defined and tailored composition.
Research Focus C.3: Ultrafast Spin Dynamics in Magnetic Nanosystems
The enormous potential of ultrafast spin manipulation for applications in information storage, processing and retrieval stimulates a growing interest in the excited states and non-equilibrium properties of magnetic nanostructures.
In ferromagnets, the exchange energy (eV) sets the characteristic time scale in the fs-regime, while demagnetization energy (meV) is the prominent interaction in the ps-regime and above. It is interesting to study confined systems where both interactions are involved. Spinwaves in confined ferromagnets are a prototype multi time-scale problem. In the integrated research project C 3.2 “Imaging dynamics of ferromagnetic nanostructures via nuclear resonant diffraction” we realize a new experimental approach to resolve spin-wave dynamics in confined geometries on sub-ns timescales with sub-nm spatial resolution.
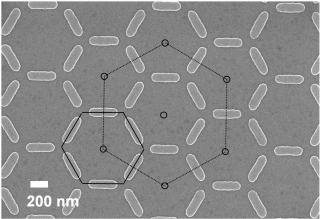
Kagome lattice nanofabricated by electron-beam lithography and lift-off processing. Each ferromagnetic nano- structure is in a quasi single-domain state.
Our goal is to investigate spin waves in frustrated lateral ferromagnetic nanostructures. Artificial spin ice has come into the focus of interest as confined ferromagnetic system. For spin ice, no single minimal energy exists and it is therefore a model system for frustration phenomena reminiscent of the water-ice systems. In geometrically frustrated single-domain magnets the dipole interaction creates a two-dimensional analog to spin ice in crystals. Static imaging of the magnetic moment of individual elements reveals the local accommodation of frustration. The so-called ice rule dictates the energetically favorable configurations although a full compensation of the local stray fields is a priori not possible. In such lattices, spin-wave modes appear prominently in the frequency spectrum of the magnetization excitation amplitude typically between ten and up to hundred GHz. The character of these spin wave excitations and their dispersion relation shall be characterized by vector-network-analyzer ferromagnetic resonance spectroscopy (VNA-FMR) and with measurements at the dynamics beamline P01 at PETRA III. The experiments are at the limit of temporal and spatial resolution of conventional magnetic microscopy, but can be realized by nuclear resonant scattering of synchrotron radiation (NRS). Time-resolved NRS is an established tool to determine the depth-resolved spin structure of magnetic thin films and nanostructures with sub-nm spatial resolution. The technique is intrinsically sensitive to time-dependent processes as magnetic switching and relaxation phenomena.
The experiments of the research project yield unique insights into topological aspects of frustrated spin systems and its dynamics. Connections to the mechanism of bond-order frustration as investigated in project C.1 for water/ice and glasses are very interesting. The second focus in C.3. is on “Ultrafast magnetic imaging of nanoscale structures” (research project C.3.2).
Participating Research Groups
- Prof. P. Alivisatos
- Prof. J. Bachmann
- Prof. G. Bester
- Prof. H. Chapman
- Prof. M. Drescher
- Prof. M. Fröba
- Prof. G. Grübel
- Prof. C. Klinke
- Prof. H. Lange
- Dr. G. Meier
- Prof. A. Mews
- Prof. R.J.D. Miller
- Prof. K. Nielsch
- Prof. H.P. Oepen
- Dr. R. Röhlsberger
- Dr. M. Schnell
- Prof. H. Weller